Defining a fetal deprivation sequence
Intrauterine growth restriction (IUGR) as a consequence of substrate deficiency has many detrimental effects on the fetus. These effects clinically present with multi-organ dysfunction. Detailed investigation of potentially affected cases was conducted in conjunction with a literature review to enable the classification of the proposed diagnosis of fetal deprivation sequence, which encompasses the presentation of infants with IUGR and multi-organ failure. This article highlights the importance of recognising this diagnosis and the impact on initial management and follow-up.
Nicola Mullins1Neonatal Consultant
nicola.mullins1@nhs.net Cath Harrison1,2
Neonatal Consultant and Transport Consultant 1Department of Neonatology, Leeds Children’s Hospital
2Embrace, Yorkshire and Humber Infant and Children’s Transport Service
It is important for clinicians to distinguish between IUGR and small for gestational age (SGA). IUGR is defined as: “A rate of fetal growth that is less than normal in light of the growth potential of that specific infant”.1 SGA has been defined as: “Having birth weight less than the 10th percentile of a population-specific birth weight for specific gestational age”.1
Growth restriction is common and is seen in 3-7% of pregnancies.2 Both morbidity and mortality are increased with perinatal mortality rates reported up to eight times higher in affected infants, and morbidities, including abnormal neurodevelopmental outcomes and cerebral palsy in 50% of surviving infants.3 More recently there has been interest in the long-term impact of growth restriction, leading to cardiovascular and metabolic disease in adulthood.4
Clinicians recognise that IUGR is associated with neonatal sequelae such as thrombocytopenia and hypoglycaemia and that this is seen following reduced fetoplacental oxygenation. However, there is decreased recognition of the impact of a lack of substrate (glucose) on organs other than the brain.
The authors had observed a cohort of babies presenting with a constellation of symptoms including thrombocytopenia, persistent lactic acidosis, hypoglycaemia, coagulopathy, and renal and hepatic failure.
Many were referred in to our centre due to liver failure. Extensive investigations were carried out to exclude a primary hepatic problem including investigations such as bone marrow aspiration to exclude causes such as haemophagocytic lymphohistiocytosis, inborn errors of metabolism and mitochondrial hepatopathies. No babies had a definitive diagnosis and, with this in mind, detailed reviews of affected infants were conducted in conjunction with a literature review to enable the classification of the proposed diagnosis of fetal deprivation sequence, which would encompass the presentation described above.
Aims
- To describe the detailed clinical sequelae of placental insufficiency by presenting the findings of multiple case reviews.
- To define a diagnostic classification for the proposed term ‘fetal deprivation’ which encompasses the presentation.
- To aid clinicians in correctly diagnosing fetal deprivation and enabling them to streamline the investigative and diagnostic approach to this challenging group of infants.
Hypothesis
There is an episode of chronic oxygen deprivation in utero, followed by a period of attempted compensation up until the time of birth, resulting in multi-system involvement but an absence of a hypoxic ischaemic encephalopathic (HIE) picture.
Methods
Over a two-year period, on a large tertiary neonatal unit, seven infants presented with hepatic failure along with signs of multi-organ failure in the absence of an acute asphyxial event. Investigation of their clinical notes and the standard neonatal database, BadgerNet, was utilised for data collection. Maternal and obstetric history was reviewed in conjunction with birth history and the clinical features identified in the affected neonates using a systems-based approach. Placental histology and neonatal outcome were also collated. The findings were then tabulated to identify common features.
Findings
All cases reviewed had a gestational age >37 weeks and had signs of IUGR due to pre-eclampsia or another maternal complication. Abnormal Doppler ultrasound examinations were apparent in two out of seven cases. Reduced fetal movements and signs of fetal distress were evident in all cases.
The need for resuscitation at birth was variable. Five cases required some support at birth involving intubation but none of the cases required cardiopulmonary resuscitation. None received therapeutic hypothermia.
Short-term ventilatory support was required immediately after birth in six cases, however, in all cases this was limited to less than 48 hours.
Cardiac dysfunction was apparent in five babies requiring inotropic support shortly after birth.
All babies had severe thrombocytopenia (platelets <30x109/L), which was resistant to platelet transfusions. Five cases had prolonged thrombocytopenia with platelet counts <80x109/L on day 7 of life.
Hepatic dysfunction presented with deranged coagulation, which was exceptionally problematic and resistant to treatment. One baby had a high inter-national normalised ratio (INR) of 7.6 on day 1 of life and this remained >4.0 despite treatment and never normalised. Six babies had an INR >1.6 on day 7 of life. An elevated alanine transaminase (ALT) level was also evident in two cases; however, a trend could not be determined. The elevation in ALT was profound with values exceeding 2,000U/L in one case.
In all cases, elevated lactate levels were seen and persisted for more than 48 hours. Where lactate levels were closely monitored, normal levels were achieved between day 4 and 6, although remaining high in the most severe cases.
Persistent hypoglycaemia was another problematic and consistent feature, with all infants requiring a minimum of 15% glucose solution to maintain normal blood glucose levels. Hypoglycaemia resolved by day 3 in all cases, except one, where normoglycaemia was never established.
Five cases had deranged renal function (defined as creatinine >88µmol/L) with the highest level seen on average on day 4 of life, although the trend was variable. Two babies had a peak creatinine >200µmol/L. Renal function normalised in only two of the affected infants. Overall, there was a high mortality with five out of the seven infants dying.
Reviewing the placental histology, three showed widespread chorionic villus infarction and diffuse histiocytic intervillousitis.
These findings have been tabulated to visually represent the key findings of the proposed diagnosis of fetal deprivation (TABLE 1).
TABLE 1 A summary of the clinical presentations.
Diagnostic criteria
In summary, our review has identified the common features of FDS as growth restriction, severe liver failure presenting with a coagulopathy, a persistently raised lactate and hypoglycaemia, often in the absence of hypoxic-ischaemic encephalopathy. The authors suggest that to fulfil a diagnosis of FDS all of the major criteria should be present with minor criteria strengthening the diagnosis if present. The suggested diagnostic criteria are proposed in TABLE 2.
TABLE 2 Proposed criteria for diagnosing fetal deprivation sequence.
Discussion
It is important to understand the underlying pathophysiology behind the presentation of FDS. Rosenberg eloquently describes the acute neonatal consequences of IUGR of perinatal asphyxia and neonatal adaptive problems.5 Our series shows that infants with FDS have a similar maladaptive process but do not present with signs of asphyxia at birth.
Harding et al describe the association of restricted fetal growth with structural and functional anomalies of the lung.6 The most common cause of fetal growth restriction is fetal malnutrition due to placental insufficiency or maternal malnutrition. In the animal model, induced growth restriction causes alterations in lung architecture with thickened alveolar membranes and fewer, but larger, alveoli leading to impaired gas exchange.7 One might assume that a similar picture exists in FDS with the chronic restriction of nutrients and oxygen and it is therefore unsurprising that infants with FDS have signs of respiratory failure requiring support after birth. Optimal nutrition in this group of infants is essential, as postnatal nutrition influences future lung development, both in terms of growth and architecture.8
It has been shown that growth restricted fetuses show signs of cardiac dysfunction.9 Substrate deprivation leads to ventricular hypertrophy and dysfunction due to increased placental vascular resistance and redistribution of the cardiac output to the left side of the heart. This abnormality persists after birth with evidence of cardiac remodelling and signs of systolic and diastolic dysfunction. Interestingly, growth restricted infants have a normal heart rate but postnatal echocardiograms show more globular hearts with higher stroke volumes.10 These findings highlight the importance of an initial echocardiogram and subsequent monitoring of cardiac function when FDS is suspected.
All of the babies in our case series showed significant and persistent thrombocytopenia. Fetal platelet counts have been described by many groups to be over 150x109/L by the end of the second trimester, remaining at this level until term.11 Severe thrombocytopenia in the newborn has been defined as a platelet count of <50x109/L and this occurs in 0.1-0.5% of babies. Like most blood cell production lines, fetal thrombopoiesis is regulated by a specific hematopoietic factor, in this case thrombopoietin (TPO), which is released at a constant rate by the liver into the bloodstream.12 Postnatal platelet production is centred on the bone marrow. If chronic asphyxia is present, the liver, along with other organs, will be subjected to a decreased oxygen supply reducing platelet production. Animal asphyxial models have shown a persistent thrombocytopenia thought to be due to a decreased rate of production of marrow megakaryocytes or a structural or metabolic defect in platelets produced under conditions of hypoxia.13 A recent review of late preterm and term neonates after perinatal asphyxia showed 31% had thrombocytopenia associated with an increase in nucleated red blood cells suggesting hypoxia was the cause.14This has been shown by other groups with increased erythropoietin levels in fetuses with severe IUGR due to chronic placental insufficiency and absent or reversed flow in the umbilical artery.15
Persistently raised lactate levels were consistently seen in our group and the levels did not normalise until four to six days after birth. It is well described that placentally restricted fetuses are chronically hypoxaemic resulting in an increased blood lactate with usually no change in arterial pH.16 Clinicians may be falsely reassured by a normal pH on fetal blood monitoring. Experimental animal data have shown that lactate is an earlier marker than pH in the hypoxic process and increases in subcutaneous tissue before pH decreases.17 Human studies suggest that lactate concentration in fetal blood samples is a better predictor than pH for neonatal morbidity.18 Growth‐restricted fetuses have poor glycogen reserves and it is possible that in pregnancies with growth‐restricted fetuses, there may be a lack of substrate to produce lactate, and thereby less increase in lactate concentrations under anaerobic circumstances. If so, the degree of lactic acidaemia in growth‐restricted fetuses might not reflect the seriousness of the hypoxia. Nicolaides et al, however, describe that growth‐restricted fetuses with intrapartum fetal distress did show an increase in lactate, with a higher median lactate concentration in SGA fetuses compared to appropriate for gestational age (AGA) fetuses, thought to be due to chronic tissue hypoxia.19 With this information, it is easy to understand why the babies in our series had such high levels of lactate.
Hypoglycaemia is highly prevalent among babies with substrate deficiency. This finding is supported by Morrison et al, who demonstrated an increase in insulin sensitivity in babies with substrate deficiency. They explain how the fetus adapts to poor nutrition by an upregulation of the insulin receptor without an up-regulation of insulin signalling in fetal skeletal muscle. However, after birth there is an up-regulation in the insulin receptor and the insulin signalling pathway resulting in a hyperinsulinaemic state causing hypoglycaemia.20 The clinical severity and duration of hyperinsulism is variable and not predictable from the severity of perinatal stress.21 Our series found the hypoglycaemia associated with FDS may be unresponsive to diazoxide and require supplemental glucose for a protracted period.
The association between substrate deficiency and impaired renal function in the short term can be explained by evidence suggesting that growth restriction reduces nephron number and function, renal circulation, reduced osmotic clearance and glomerular filtration rate, albeit in an animal model.22-24 Renal impairment manifests later on in life due to the effects of type 2 diabetes and hyper-tension associated with previous IUGR.25
Liver malfunction with elevated ALT and a coagulopathy was a prominent feature in this case series. Liver cell injury is common after perinatal asphyxia and presents with elevated ALT, alkaline phosphatase (ALP), aspartate transaminase (AST) and lactate dehydrogenase (LDH). In an acute event this derangement is transient.26 However, in the context of chronic ischaemia, seen with fetal deprivation sequence, ischaemic hepatitis can occur due to hepatocyte hypoperfusion.27 This results in the liver being unable to synthesise blood clotting proteins leading to a coagulopathy.
As highlighted previously, immediate brain dysfunction in the form of HIE is not clinically or electrically apparent in this cohort of patients. The majority of babies in our cohort had normal cranial ultrasound scans (USS) and normal neurology. This finding is supported by the known adaptive fetal response, which preserves brain oxygen supply during chronic hypoxia. However, data suggest that this mechanism may not compensate for aberrant cellular successions resulting in impaired neurodevelopmental outcome.28 Magnetic resonance imaging (MRI) studies on infants subject to IUGR have identified a reduction in frontal lobe volume relative to intracranial volume, potentially affecting later development of complex executive functions such as cognition, behaviours and language. The hippocampus also appears affected with a 30% reduction in hippocampal neurone development.29
These data highlight the importance of developmental follow-up in babies with suspected FDS as the above findings would not be detected on cranial USS alone.
Our case series has highlighted the variable practice in obtaining placental histology (three out of seven cases with histology). However, it has become apparent that placental histology can be extremely helpful towards supporting a diagnosis of FDS due to the common histopathological features present with IUGR. These include villous infarction, elevated nucleated red blood cells, increased syncytial knotting, thrombi or haematomas and villitis. Absent or reduced end diastolic flow in the umbilical artery has also been shown to increase the numbers of nucleated red blood cells and distal villous hypoplasia.30 However, due to the time constraints in obtaining detailed histological investigations, placental histology is unlikely to be beneficial acutely but alongside other investigations, supports the diagnosis of FDS.
Clinically, babies subject to chronic hypoxia and substrate deficiency can be difficult to manage. The challenge is finding the right balance in terms of investigations and ongoing management as mortality rates are high. The authors suggest that if the diagnosis of FDS seems likely then a targeted investigative approach to ascertain organ function is appropriate. An echocardiogram at initial presentation, and as part of ongoing monitoring, is recommended to ascertain cardiac function due to the association of ventricular hypertrophy and dysfunction. Placental histology should be carried out in cases with clinical features of FDS.
Long-term follow-up of these infants also needs to be considered. At present, babies who fulfil the criteria of FDS seem neurologically unaffected with a normal cranial USS. As a consequence, survivors are often not considered for long-term follow-up. However, as the evidence documented here highlights, initial normal brain imaging should not be considered reassuring. These babies should be considered as high risk and thus fulfil the National Institute for Health and Care Excellence (NICE) guidance for two-year and four-year follow-up. An MRI may be warranted at the two-year follow-up, dependent on developmental progress.
Medico-legally the classification of FDS is relevant. Presentations similar to those described here are often attributed to severe late fetal hypoxia when in fact they are a consequence of a different more chronic entity, which has been termed the fetal deprivation sequence by the authors.
Conclusion
Our review shows that babies affected by FDS have a similar clinical picture of multi-organ failure but with relative ‘brain sparing’ due to fetal substrate deprivation. Clinicians need to take a detailed history including fetal wellbeing parameters and discussion with obstetricians to ascertain the presence of signs of FDS. Since collating this data, the authors have recognised this pattern locally in over 20 more term babies, all fulfilling the criteria suggested for this diagnosis.
A diagnosis of FDS in the clinicians’ differential helps rationalise investigations and management of these babies. Additionally, being mindful of this diagnosis will result in a more thorough approach to follow-up including postnatal counselling for families with affected infants.
Author contribution
Both authors contributed equally in the data collection, analysis and writing of this paper.
Acknowledgement
The authors wish to acknowledge the late Dr Simon Newell for his support and belief in this work.
Or read this article in our
Tablet/iPad edition
- IUGR as a consequence of substrate deficiency causes multi-organ failure often with ‘brain sparing’.
- The term fetal deprivation sequence (FDS) is suggested to describe the typical presentation of these affected babies.
- Diagnostic criteria for FDS have been defined to help rationalise investigations and management, and guide follow-up of these affected babies.
Also published in Infant:
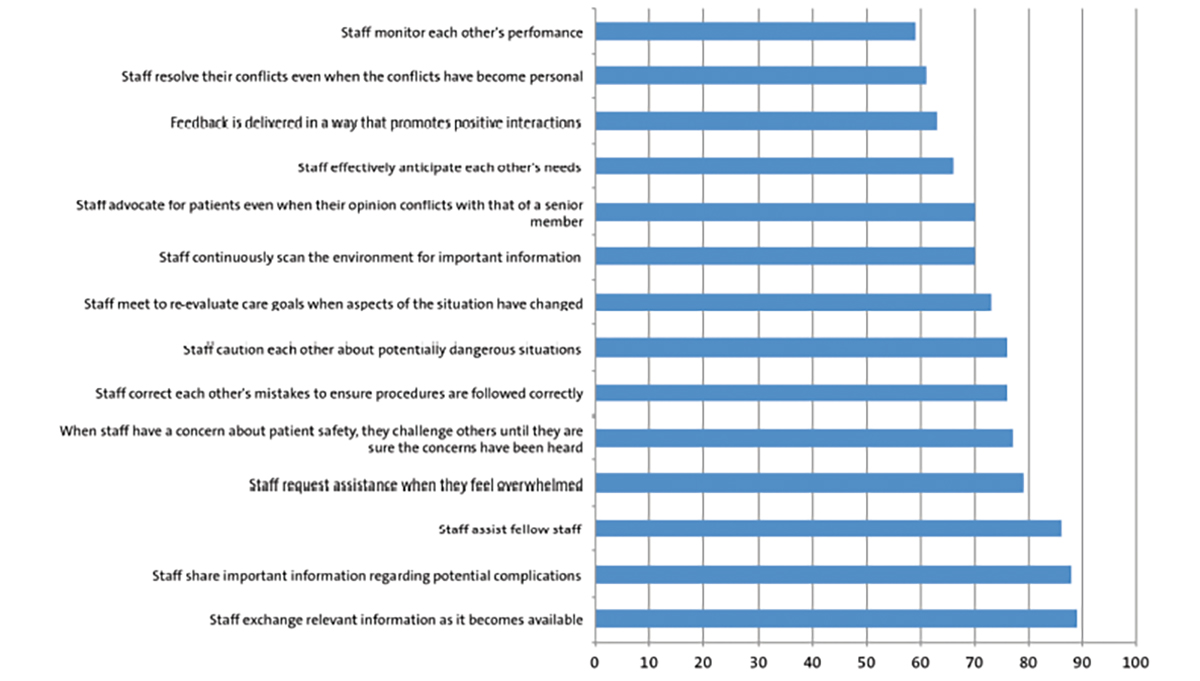